LIDAR systems are a vital technology for the future of automation. Along with cameras and radar sensors, LIDAR systems can detect orientation, obstacles, and distances to improve the safety and efficiency of automated systems. Optics for LIDAR systems are a crucial component of creating a system with optimal performance.
What Is LIDAR?
LIDAR stands for Light Detection and Ranging. It’s a remote-sensing method that uses light in the form of a pulsed laser to measure ranges to the Earth.[1] Along with other data recorded, LIDAR can generate precise, three-dimensional information about the shape of the Earth and its topography.
LIDAR instruments consist of a laser, a scanner, and a specialized GPS receiver. Commonly used in airplanes and helicopters, LIDAR may be topographic and bathymetric.
Topographic LIDAR typically uses a near-infrared laser to map the land. Bathymetric LIDAR uses water-penetrating green light to measure the seafloor. This allows scientists to examine environments with accuracy and precision.[1]
Compared to traditional photogrammetric approaches, LIDAR is less sensitive to the time of year, time of day, or current weather patterns in which the data was collected. It can also generate high-resolution 3D surface information more rapidly because of its ability to penetrate vegetation and other obstructions.
In addition, LIDAR is collected from ground-based stationary and mobile platforms for street mapping and autonomous driving applications. Engineers and surveyors use LIDAR to develop railroads, bridges, roadways, buildings, breakwaters, and shoreline structures.[1]
Principles of LIDAR Data Collection
An airborne LIDAR system uses a laser scanner unit, a GPS, and an IMU. The laser scanner has a laser range finder unit and a beam deflection device to create a scanning pattern. The GPS delivers the position of the sensor platform, and the IMU records the angular altitude. Together, these allow the system to provide the aircraft’s absolute position, which is then synchronized using the detector’s recording system.
LIDAR has several advantages as a remote sensing technique, including high accuracy, extensive coverage area, and large point density. Engineers and surveyors can resample the regions quickly and efficiently as well.
Laser Requirements for LIDAR
The laser is a significant component of a LIDAR system. Because of this, the laser specifications determine the application’s overall performance, cost, and viability.
- Laser wavelength: The wavelengths used in LIDAR systems include 1064 nm, 532 nm, and 1550 nm. Each wavelength offers pros and cons that affect performance.
- Pulse repetition rate: The pulse repetition rate and pulse energy determine the sampling rate of a LIDAR system.
- Efficiency and weight: LIDAR systems are typically mounted on aircraft, whether piloted or unmanned craft or drones. As a result, the laser source must be compact, lightweight, and efficient.
- Laser pulse width: The laser pulse width determines the vertical target resolution or range. Current LIDAR sensors use laser pulse widths in the 2-5 ns range.
- Laser power and beam divergence: The maximum distance that data can be measured is vital to LIDAR end users.
- Spectral width: LIDAR systems are typically 1064 nm wavelength for the laser availability and high reflectance of targets.
LIDAR Applications
LIDAR is necessary for many industries, including semi-autonomous and autonomous vehicles and law enforcement.[2]
Here are some other applications of LIDAR:
Agriculture
LIDAR has many applications in agriculture. It can be used to increase yields and use land more efficiently with real-time monitoring in precision farming. It can also pinpoint areas with optimal sunshine for more efficient growing or crops that need water. Another application in agriculture is for drones, which map agricultural areas and natural resources using geospatial measurement data obtained by LIDAR.
Archaeology
LIDAR offers clear mapping to pave new ways for archaeology. Building ruins and artifacts that were previously undiscoverable due to vegetation can now be imaged and mapped in broad detail using LIDAR. Archaeologists can also discover hidden structures in remote environments using drones with LIDAR, which can scan roughly 600 acres of land each day.
Astronomy
Astronomers can use LIDAR technology to create topographical maps of Mars and other planets. It can be used to measure wind, map surface topography, roughness, tidal deformations, and other geodetic properties of distant plants that are otherwise unexplored.
Land Mapping
NOAA uses LIDAR to create shoreline maps and digital elevation models or to plan emergency response missions. Both topographic and bathymetric LIDAR offer significant mapping capabilities in land and over aquatic environments for surveying, allowing companies to create 3D terrain models.
Energy
LIDAR has a wide range of applications in energy infrastructure. Renewable energy sources, oil and gas resources and resource mining can be obtained using 3D elevation data to assess sites for energy infrastructure, such as refineries or pipelines, and mitigate the risks from natural disasters. It may also be used to scan oil and gas infrastructures from the air, quantify methane emission rates, and improve safety.
Meteorology
LIDAR is used in meteorology to measure atmospheric constituents, such as pollutants and other particles, and their dynamics. Analyzing this information is important for forecasting and monitoring atmospheric conditions in real-time, which is important for advanced warning in the case of natural disasters like tornadoes, thunderstorms, and flooding. LIDAR provides an essential “middle ground” between near-ground data and satellite and numerical data.
Robotics
LIDAR is essential for assisting robots in navigation. LIDAR enables object perception, identification, and collision avoidance to help robots understand their environments and operate autonomously. This is vital to many industries increasing the use of autonomous or semi-autonomous robots, including industrial automation, security, deliveries, warehouse logistics, and research.
The Role of Optics in LIDAR
Though many components make LIDAR work, optics are among the most important. Generally, the bigger the optics in a LIDAR system, the better it can perform. Optics collect signal without collecting more noise.
The key challenges for optics in a LIDAR system include projecting the beam as far as possible without affecting eye safety and collecting as many photons as possible. This requires the most uniform, outgoing beam with no distortion – a difficult task all around.
Obtaining a uniform beam (ideal collimation) is challenging. Different types of lasers produce different patterns, which need to be adjusted to transform into a uniform parallel beam. Typically, this requires multiple optical elements working together.
The lenses need to be the optimal size to get clear imaging and an efficient collection of photos, which is a balance of the different parameters of engineering to avoid compromising one in pursuit of better performance from another.
Striking this balance involves some key considerations:
- Material choice
- Lens coatings
- Configuration
- Receiver
Optics for LIDAR Systems from Apollo Optical Systems
Apollo Optical Systems produces the highest quality polymer optical components to meet the demanding needs of LIDAR systems, including flat windows, curved or cylindrical windows, domes, reflectors, imaging optics, and prisms.
Polymer optics can reduce the size and weight of your optical system or provide complex optical surfaces. Apollo Optical Systems offers optics manufacturing with mounting features like slots and pinholes directly into the component for a lightweight and efficient device. Contact Apollo Optical Systems to discuss your custom LIDAR optics project!
Sources:
[1] https://oceanservice.noaa.gov/facts/lidar.html [2] https://www.americangeosciences.org/critical-issues/faq/what-lidar-and-what-it-used

About Dale Buralli
Dr. Dale Buralli has served as the Chief Scientist for Apollo Optical Systems since 2003. In this role, Dr. Buralli is responsible for the design and optical modeling of various optical systems. These systems include virtual or augmented reality, ophthalmic and other imaging or illumination systems. Additionally, he provides support for optical tooling of lens molds and prototypes, including the development of custom software for both production and metrology. Dr. Buralli got his Ph.D. in optics from the University of Rochester in 1991. Now he is an Adjunct Professor of Optics at the University of Rochester’s Institute of Optics.
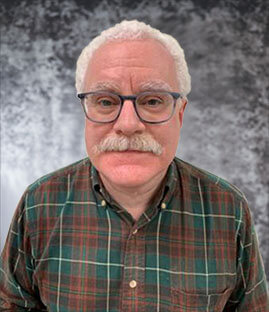